Structural Heterogeneity
One of the greatest challenges to determining the structure of protein complexes is that many exist not in a single structural form, but rather as an ensemble of states. Traditional structural biology approaches are inherently 'averaging', in that they provide a view over all molecules in solution. Mass spectrometry, in contrast, is inherently separative, and thereby allows the interrogation of the individual co-populated states. We are exploiting this benefit to identify the different protein stoichiometries present in solution, and to quantify their relative abundance. Moreover, by employing ion mobility spectrometry we can obtain size measurements for the different assemblies, which we can use to distinguish between structural models. This approach is particularly beneficial when used in combination with information from other low-resolution methods, as we are doing in combination with transmission electron microscopy.
Protein Dynamics
Protein complexes are not static structures, and therefore in order to understand properly their function on the molecular level we need to consider the motions they undergo. Such fluctuations are however very challenging to study, and for the majority of proteins with known structures the complementary dynamical information is lacking. We have developed mass spectrometry means for quantifying the changes in quaternary organization of protein assemblies by monitoring the rate at which they exchange subunits in real time. Where possible we combine the mass spectrometry results, which report on the oligomer level, with measurements of dynamics on the level of the individual amino acids as provided by techniques such as nuclear magnetic resonance or fluorescence. We are interested to investigate whether variant proteins display compromised dynamics, and whether this might provide a rationale for their involvement in human disease.
Molecular chaperones and protein homeostasis
We are interested in ‘molecular chaperones’, proteins which act to prevent or reverse prevent improper polypeptide associations. Molecular chaperones thus play a vital role in protein homeostasis, the mechanism through which the cell maintains the proteome by balancing the influence of a multitude of biochemical pathways. The breakdown of this balance can lead to a variety of diseases, such as Alzheimer’s, type II diabetes, and certain cardiomyopathies, many of which are characterised by the deposition of misfolded proteins. We are applying our ability to disentangle equilibrium heterogeneity and fluctuations to overcome the complexity of the proteostasis network, and thereby characterise the structure, dynamics, and interactions of molecular chaperones and their targets.
Small Heat-Shock Proteins
A wide-spread family of molecular chaperones are the Small Heat-Shock Proteins (sHSPs), which act to capture destabilised target proteins, preventing their aggregation and insolublisation. sHSPs are therefore the ‘paramedics’ of the cell, on the front line against the deleterious consequences of protein unfolding, and their malfunction is consequently implicated in a range of human pathologies. The sHSPs have long been regarded notorious challenging targets for structural biology, due to their flexibility, dynamic fluctuations at equilibrium, and frequent heterogeneity. These properties combine to create a perfect storm, which we have shown mass spectrometry based approaches are well-equipped to weather. We are interested in many aspects of the sHSPs: their atomic details; regulation; and function in health and disease.
Tuning the self-assembly of proteins
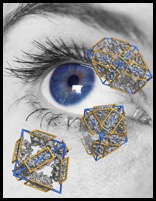
Controlling the self-assembly of materials into structures on the nanometre scale is a frontier challenge of engineering and physical sciences. The widespread development of such materials however has been significantly hampered by a combination of both deficiencies in the theoretical descriptions of the interactions that dictate the structures, and the experimental tools available to probe these structure features. Many of the most striking examples of self-assembly are found in biology, governed by a myriad of different types of interaction. Indeed the majority of proteins in the cell are actually found to be natively assembled into specific oligomeric complexes. Some of these assemblies, including viral capsids, components of the protein folding and degradation machinery, and metal-storage proteins, have distinctive cage-like structures. In nature these protein nano-cages act to sequester important and sensitive cargo from the surrounding environments, providing inspiration for exploiting this in synthetic applications. We are exploring the potential of the sHSPs as tuneable nano-cages, ascertaining the diversity in both spatial and temporal dimensions these molecules have evolved and how they can be manipulated to direct self-assembly.
Mass spectrometry for structural proteomics
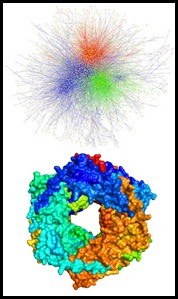
In order to capture the diversity in structure and dynamics displayed by proteins and other biomolecules it has become apparent that no single technique can capture all the details. As such there are great benefits of combining approaches, both experimental and computational, to obtain a more complete description of the system. Nonetheless challenges in performing this remain: information from different techniques needs to be correlated, or translated into common spatial restraints; and meaningful ways of weighting the ‘hybrid’ data-sets are required. It nonetheless also remains important to maximise the information from each approach, and the application of mass spectrometry approaches remains under-explored. We are developing experimental and data analysis methodologies to address this gap. Extension of these approaches provides a means for MS-based ‘structural proteomics’. Furthermore we have established means of combining spatial and dynamical MS data with information from complementary technologies including electron microscopy and NMR, respectively. Exploiting these synergies promises the development of hybrid approaches capable of providing novel insight into proteins otherwise resistant to molecular characterisation.